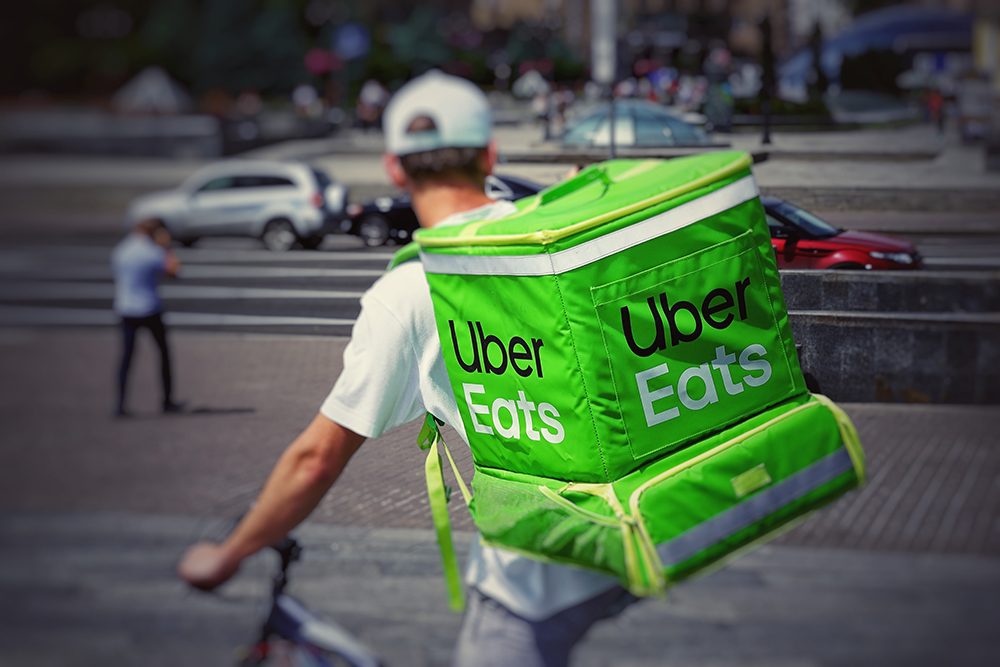
When a planet transits in front of its parent star, some of the light is not only blocked, but if an … [+] atmosphere is present, filters through it, creating absorption or emission lines that a sophisticated-enough observatory could detect. The best current limits have revealed only Saturn-sized atmospheres around Sun-like stars and Neptune-sized atmospheres around red dwarfs, but James Webb will get us super-Earths.
ESA / David Sing
Over the past 30 years, we went from not knowing if there were planets like ours around other stars to a catalogue containing thousands of them. Today, we know of over 4,000 confirmed exoplanets, with a few of them even possessing properties that we assume might be friendly to life. However, the most typical planet found by NASAs Kepler mission isnt exactly like anything found in our Solar System, but rather has a mass and size somewhere in between the size of Earth and Neptune. Are they more like Earth, with surfaces and thin atmospheres, or like Neptune, with large, volatile gas envelopes? Thats the burning question on Dr. Xinting Yu, a postdoctoral fellow at UC Santa Cruz, who writes in to suggest a new way of looking at a longstanding problem:
We are publishing a new article on detecting solid surfaces or liquid oceans on exoplanets… none of the upcoming space telescopes have the capacity to “see” the surface of the exoplanet directly, but they are excellent at seeing the atmospheric composition. I am sending you this paper in case you are interested!
I did take a look, and not only am I interested, but I think everyone will be really excited about this upcoming technique that might, for the first time, tell us which exoplanets in the so-called super-Earth category really do have surfaces, rather than volatile gas envelopes. Heres how.
When Mercury (upper) first begins transiting across the Sun, there is no hint of an atmospheric … [+] ‘arc’ that would reveal the presence of sunlight filtering through its atmosphere. By contrast, Venus’ atmosphere (lower) displays a clearly defined arc during transits, and did as far back as the 18th century. Transits have the potential to reveal an atmosphere’s presence, composition, and thickness, even for exoplanets.
NASA/TRACE (top); JAXA/NASA/Hinode (bottom)
The problem is as follows. The way weve discovered the overwhelming majority of our exoplanets the planets found in space that orbit stars beyond our Sun is through the transit method. You can imagine two possibilities for what it would look like to watch the planets orbit our Sun from far away:
- either we see the planets orbit the Sun at a sufficiently large angle so that they never crossed in front of or ducked behind the Sun from our perspective,
- or the orientation of the planetary orbits would be almost, or even perfectly, edge-on, so that some, or possibly even all of the planets, eventually and periodically crossed in front of or ducked behind the Sun.
That second option is rare, of course. But given that NASAs Kepler mission was watching the same patch of sky, viewing over 100,000 stars at once for a period of ~3 years during its primary mission, its no surprise that we would up revealing thousands of stars with planets around them. Not only that, but many of those stars had multiple planets, with one system (at least) containing at least as many as our own, with eight discovered so far.
This figure shows the number of systems with one, two, three, planets, etc. Each dot represents one … [+] known planetary system. We know of more than 2,000 one-planet systems, and progressively fewer systems with many planets. The discovery of Kepler-90i, the first known exoplanet system with eight planets, is a hint of more highly populated systems to come.
NASA/Ames Research Center/Wendy Stenzel and The University of Texas at Austin/Andrew Vanderburg
From the transit method, a fraction of the stars light will be blocked periodically by the planet: every time the planet passes in front of the stars disk. Because astronomers understand how both stars and gravitation work, we can infer the physical size (like the radius) of the planet, as well as its orbital properties as it revolves around its parent star.
If we then follow up our transit observations with a radial velocity study where we measure how the star gently appears to periodically move towards us, then become stationary, then move away from us, then become stationary, then towards us again, etc. we can even learn the mass of the orbiting planet. With those three pieces of data:
- the mass of the planet,
- the size of the planet,
- and the orbital distance of the planet from the star,
we can start to think about the most burning question that astronomers who study these exoplanets have in their minds: which of these planets, if any, might be suitable for life? And, if were very, very lucky, might any of them actually be inhabited?
Although more than 4,000 confirmed exoplanets are known, with more than half of them uncovered by … [+] Kepler, finding a Mercury-like world around a star like our Sun is well beyond the capabilities of our current planet-finding technology. With the enormous numbers of super-Earths we have, however, knowing which are Earth-like and which are Neptune-like becomes vitally important.
NASA/Ames Research Center/Jessie Dotson and Wendy Stenzel; missing Earth-like worlds by E. Siegel
We know, from both our own Solar System and the observations weve made around other stars, that some exoplanets are very, very likely to be rocky planets similar to the ones we find in our own neighborhood: Earth, Venus, Mars, and Mercury. They might be airless like Mercury, have very thin atmospheres like Mars, have life-and-water-friendly atmospheres like Earth, or have substantial, but not gas giant-like, atmospheres like Venus.
Weve seen, based on the densities of many worlds, that the overwhelming majority of planets with masses below 2 Earth masses and radii below about ~1.2 Earth radii are, in fact, rocky like the ones in our own backyard.
Similarly, we can tell with a large degree of certainty that if you have more than about 10 Earth masses, or more than about ~2 Earth radii, youre almost certainly going to be more like Uranus or Neptune: holding on to a large, massive envelope of hydrogen and helium gases. Theres probably a surface somewhere down there, but youd have to go down below more than ~1000 times the atmosphere currently present on Earth, rendering you more like a gas giant.
If your exoplanet is below 2 Earth masses, you’re almost certainly a rocky planet. If your exoplanet … [+] is above about 15 Earth masses, you’re almost certainly a Neptunian world. But in between? We’d have to measure to know for sure, as there’s likely variability among which planets are super-Earths versus mini-Neptunes.
Chen and Kipping, 2016
Somewhere, larger than Earth but smaller than Neptune, is a transition point, where planets, on average, are no longer able to maintain a thin atmosphere with a potentially habitable surface beneath them, and instead successfully hang onto the volatile gases that were around during the early phases of the Solar System. Knowing which worlds are rocky, with thin atmospheres, is an important key to identifying the first worlds beyond our Solar System to search for extraterrestrial life.
The problem is that, despite all our advances in finding, characterizing, and understanding exoplanets, there are still relatively few of them that are small enough and low enough in mass to definitely be rocky. Moreover, even a smaller subset of those are likely to be habitable, as most of them are either too hot or too cold to potentially house liquid water on their surfaces.
However, what we presently call super-Earth planets are actually the most common type of exoplanet found by NASAs Kepler mission. If some, most, or all of these in-between planets actually turn out to have solid surfaces with thin atmospheres, they could revolutionize the search for life beyond Earth.
Left, an image of Earth from the DSCOVR-EPIC camera. Right, the same image degraded to a resolution … [+] of 3 x 3 pixels, similar to what researchers will see with future exoplanet observations. Even if we could only get a single pixel measurement of a planet like Earth, we’d be able to pull out a wealth of scientific information.
NOAA/NASA/Stephen Kane
In an ideal world, wed have a telescope that was capable of directly imaging these exoplanets: seeing and measuring their own emitted/reflected light directly. If we had a large enough, sensitive enough telescope that could successfully block out enough of the parent stars light while still letting the orbiting planets light through, it would give us a wonderful way to answer that question directly. Even if an exoplanet only showed up as a single pixel in our telescopes, that point of light would change in important ways over time. With enough data, we could infer:
- how quickly the planet rotated on its axis,
- whether it had total or partial cloud cover and what the composition of those clouds was,
- whether it had continents and liquid oceans and what fraction of the world was covered in water,
- whether it had polar icecaps that grew and shrank with the seasons, teaching us about the planetary climate,
- whether the colors of the continents greened-and-browned, or otherwise changed with the periodic seasons,
and many other fascinating pieces of data. Unfortunately, we do not yet know whether the one telescope thats been proposed thats capable of making those observations NASAs concept flagship mission currently under review, LUVOIR will be selected to be built and launched.
If the Sun were located 10 parsecs (33 light years) away, not only would LUVOIR be able to directly … [+] image Jupiter and Earth, including taking their spectra, but even the planet Venus would yield to observations. Direct imaging of exoplanets would be the most surefire way to characterize their surface properties.
NASA / LUVOIR concept team
But whether it is or not, we dont want to have to wait until more than a decade from now to find those answers. Direct imaging of these worlds might not be on the immediate horizon, but NASAs James Webb Space Telescope, slated to launch later this year, can teach us about an exoplanets composition in a different way: through what we call transit spectroscopy.
When an exoplanet passes in front of its parent stars disk, most of that light gets blocked by the disk of that planet. But just like the Moon turns red during a lunar eclipse, because sunlight filters through the Earths atmosphere, red more efficiently than blue, and lands on the Moon a small portion of the light that gets through will have certain wavelengths of light absorbed more than others.
By breaking the observed starlight during a transit into its individual wavelengths, and then comparing that with the spectrum of the star while there is no transit, we can measure the relative atmospheric contents of whatever gases we like: oxygen, nitrogen, methane, ammonia, water vapor, carbon dioxide, etc.
An artist’s illustration of a world that would be classified as a rocky super-Earth. When a planet … [+] transits in front of its parent star, a fraction of that starlight filters through the atmosphere, exciting the emission of certain wavelengths and having light absorbed at others. The absorption spectra should provide a wealth of information about transiting exoplanets above a certain size.
ATG medialab, ESA
The bigger your planet is relative to the star, the more light its going to block, and the easier it is to detect its atmospheric signatures. We dont think that NASAs James Webb Space Telescope wont be able to measure the atmosphere of Earth-sized planets around Sun-like stars, but it should be able to measure super-Earth atmospheres around Sun-like stars.
It’s going to be very difficult to know if an exoplanet is inhabited, as all we expect to get from these indirect measurements are “hints” towards the possible existence of life. However, the question of whether the exoplanet we’re looking at has a surface or not whether it’s a super-Earth or a mini-Neptune might be answerable as soon as the James Webb Space Telescope observes it.
The key insight which the new paper details came from thinking about the atmospheres of two very, very different worlds in our own Solar System: Jupiter, the largest planet of all, and Titan, the giant moon of Saturn, which is the only moon in the Solar System with a thicker atmosphere than Earths.
High up in the atmosphere of a planet, photochemical reactions occur. If the planet has a deep … [+] surface and a large temperature gradient, the denser species will sink down to the bottom while the hotter, less dense species will rise, replenishing the dissociated molecules. If the planet has a shallow surface, however, the photochemical reactions can proceed to completion. This should lead to different abundance ratios depending on the surface depth of the planet.
X. Yu et al., arXiv:2104.09843
Think about one simple molecule: ammonia, which is nitrogen-based. Both Jupiter and Titan have small but detectable amounts ammonia in their atmospheres. In the upper atmospheres of both worlds, photochemical reactions from the Sun destroy ammonia, creating nitrogen gas and hydrogen. If you look at Jupiter, you see almost no nitrogen gas but plenty of hydrogen and ammonia, while if you look at Titan, you see plenty of nitrogen gas but almost no hydrogen or ammonia.
Why?
Because Jupiter has a thick atmosphere, and the deeper down you go, the hotter it gets. The denser nitrogen can sink down to the lower layers, while the lighter volatiles can rise up and repopulate the upper atmosphere. Meanwhile, Titan has a thin atmosphere, meaning that the temperature gradient between its surface and upper atmosphere is small. Over time, the ammonia gets depleted and not replaced, leaving the nitrogen to just hang out. By measuring the ratios of something as simple as nitrogen to ammonia, we can determine, from photochemical modeling, whether theres a thin atmosphere and hence, a surface or an atmosphere so thick that theres no evidence for a surface at all.
The differing mixing ratios of various species of molecules depends on atmospheric pressure. By … [+] measuring those ratios directly for multiple interrelated species of molecules, which the James Webb Space Telescope will be able to do, it should be possible to infer what the pressure/depth of the atmosphere is.
X. Yu et al., arXiv:2104.09843
It turns out, according to this novel scientific result, that it isnt just ammonia/nitrogen thats sensitive to the existence of and depth to the planetary surface below. Other molecules methane, ethane, water, carbon dioxide, carbon monoxide can also be present, allowing for interesting molecules (like hydrogen cyanide) to form where multiple species existed initially.
Just by measuring the chemical composition of the upper atmosphere of an exoplanet, which we can do for many so-called super-Earth worlds with James Webbs capabilities, we should be able to learn how thick its atmosphere is. Whether it has a shallow surface (like Earth), an intermediate surface (like Venus), or a deep surface (like a gas giant) will all govern the ratios of gas that well observe.
These are observations that the James Webb Space Telescope can make immediately upon beginning science operations, and it could tell us even though its indirect information which of these larger-than-Earth exoplanets really are super-Earths, with shallow atmospheres and nearby surfaces, and which ones have atmospheres that are so deep that their surfaces are all but undetectable.
This flowchart shows how molecular abundance measurements lead to surface characterization. If … [+] ammonia and hydrogen cyanide fractions are large, we have a deep surface. If they’re small, measuring various hydrocarbon ratios can tell us whether we have a shallow (Earth-like) or intermediate (Venus-like) atmosphere. At last, we’ll be able to know if these larger-than-Earth planets are super-Earths or mini-Neptunes.
X. Yu et al., arXiv:2104.09843
Ever since the first exoplanets were discovered, the ultimate dream has been to find those cosmically rare worlds like our own: the ones where life exists. As our technology progresses, we can begin to measure properties of these worlds that help us understand how suitable for life they are. At present, we can know their mass, radius, and orbital parameters, but we cant tell if they have surfaces, thin or thick atmospheres, or suitable conditions for life.
With the James Webb Space Telescope and the transit spectroscopy technique, however, we can take an enormous leap forward: we can determine which of these larger-than-Earth exoplanets are mini-Neptunes with enormous, gaseous envelopes, and which ones really are super-Earths, with thin atmospheres and solid surfaces.
In the quest for life beyond Earth, every piece of information matters. Remarkably, a novel study has shown that just by measuring the atmospheric concentrations of various species of gas something that James Webb will be able to do we can learn, at long last, if any of the exoplanets weve discovered really are super-sized versions of Earth.
Send in your Ask Ethan questions to startswithabang at gmail dot com!